All you need to know about wafering blades (understanding variables & specifications)
This guide is designed to help you navigate the various variables and possibilities associated with diamond wafering blades, crucial for precision sectioning and sample preparation. By gaining a deeper understanding of these variables and principles, you will be better equipped to identify which factors are most relevant to your specific metallography or sample preparation needs. This knowledge will enable you to make informed decisions about blade selection, ensuring optimal results and efficiency in your cutting operations.
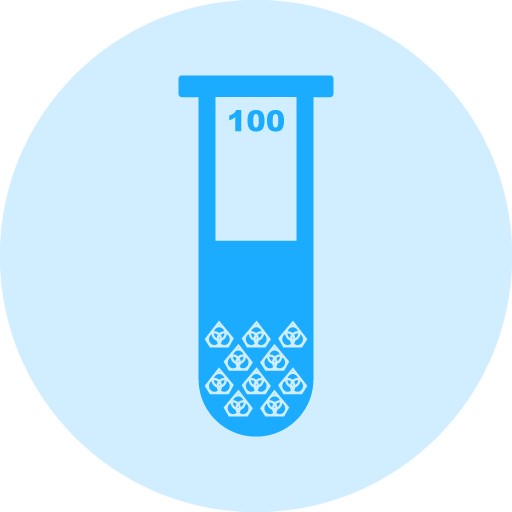
Diamond Concentration
Selecting the right diamond concentration is not merely a matter of preference but a strategic decision based on material characteristics, desired outcomes, and operational conditions. Whether the priority lies in speed, precision, cost-efficiency, or blade longevity, understanding the role of diamond concentration can greatly enhance the effectiveness and efficiency of your sectioning (cross sectioning) operation. This knowledge empowers users to make informed choices that align with their specific needs in material sectioning and sample preparation.
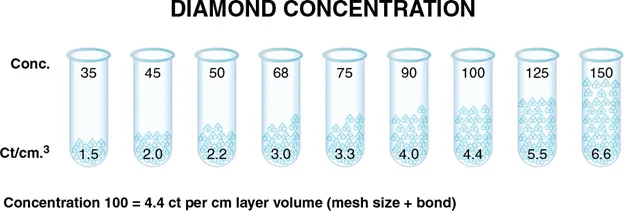
Understanding Diamond Concentration
Diamond concentration refers to the amount of diamond particles embedded within the matrix of the blade's edge. This concentration is a critical factor that influences both the lifespan and the performance of a diamond sectioning or wafering blade. The choice of diamond concentration should be tailored to the material properties and the desired cutting dynamics.
Applications and Advantages of Low Diamond Concentration
For cutting ultra-hard and brittle materials, such as advanced ceramics, glass, and certain composite materials, blades with a low diamond concentration are often preferred. In these blades, the distribution of diamond particles is sparser, which means that each diamond experiences more pressure during the cutting process. This high pressure facilitates a micro-fracture mechanism, where the diamonds effectively chip off small flakes from the material being cut. This method is particularly effective for brittle substrates, where the precision of the cut and the minimization of material damage are paramount.
Benefits of Low Diamond Concentration:
-
Enhanced Precision: Fewer diamonds mean less chance for erratic cuts, providing cleaner and more controlled sectioning.
-
Reduced Heat Generation: Sparse diamond distribution can reduce friction and consequently heat, which is beneficial for materials sensitive to thermal stress.
-
Cost Efficiency: Less diamond content can reduce the initial cost, although this may affect the blade's longevity and speed for certain materials.
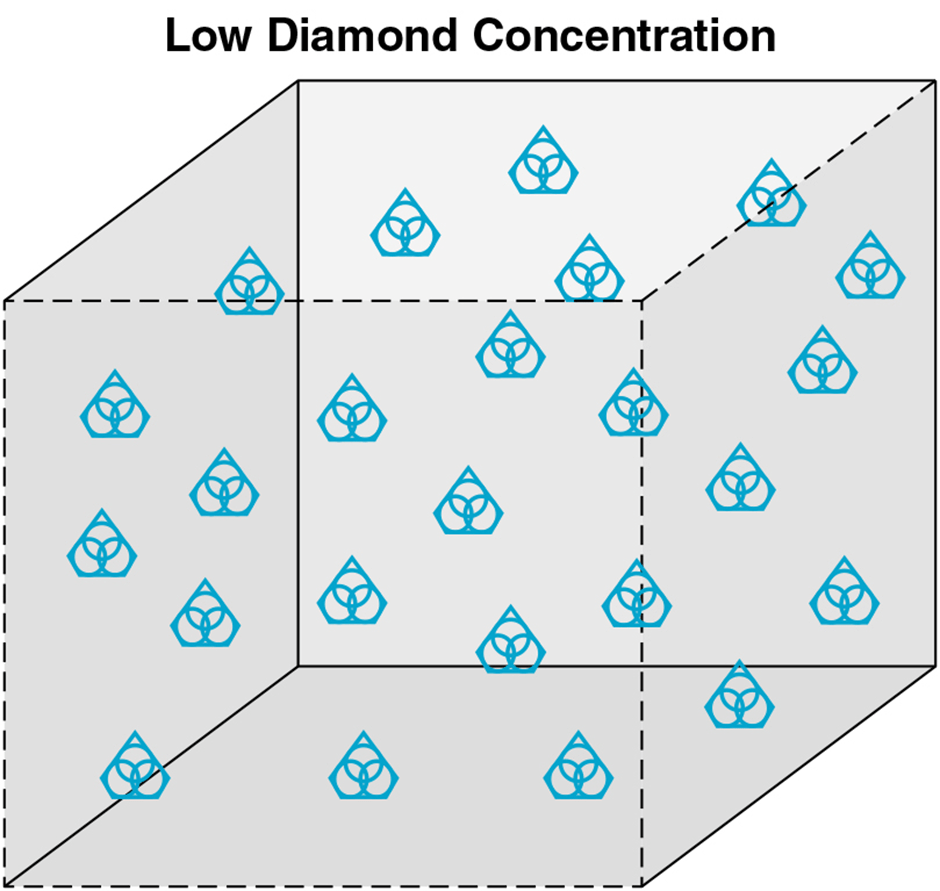
Applications and Benefits of High Diamond Concentration
Conversely, high diamond concentration blades are tailored for softer, more abrasive materials such as metals, plastics, and polymers. These materials require a blade that can endure extensive wear and tear while maintaining a fast cutting speed. The dense distribution of diamonds within the blade matrix allows for a “plowing” cutting mechanism, where the diamonds push through the material, causing the material to harden and then break away in small pieces.
Advantages of High Diamond Concentration:
-
Increased Cutting Speed: A higher concentration of diamond particles can accelerate the cutting process by intensifying the material removal rate.
-
Extended Blade Life: The abundant presence of diamonds reduces wear on individual crystals, thereby extending the blade’s operational lifespan.
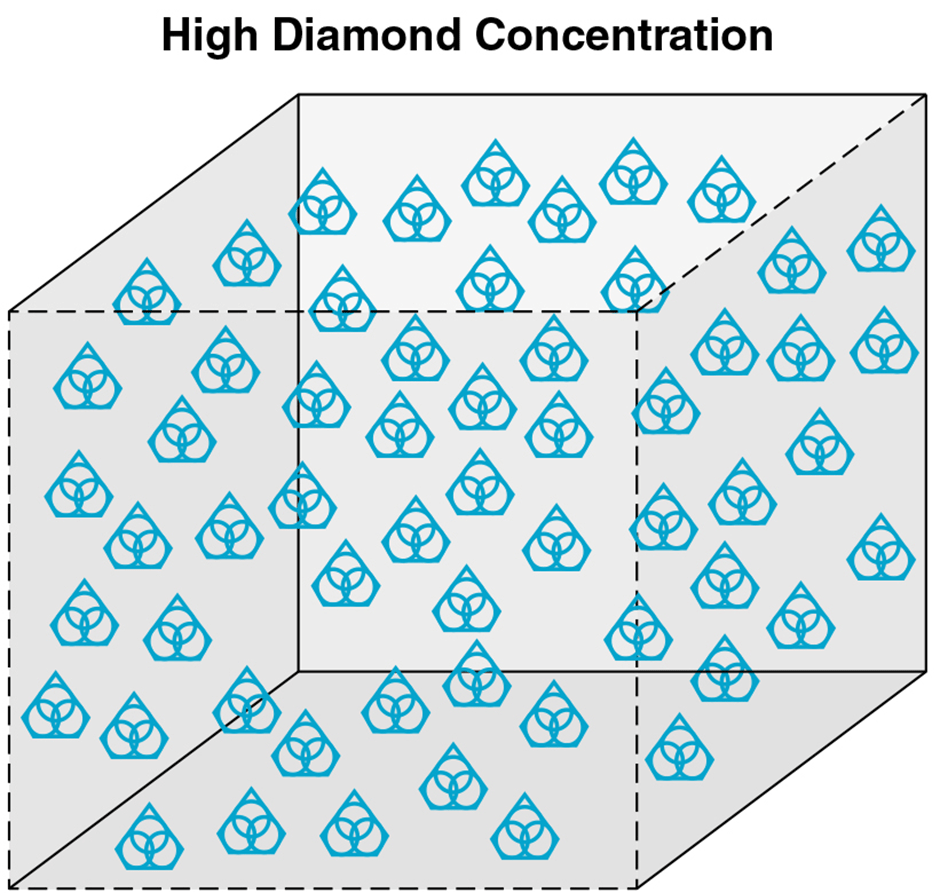
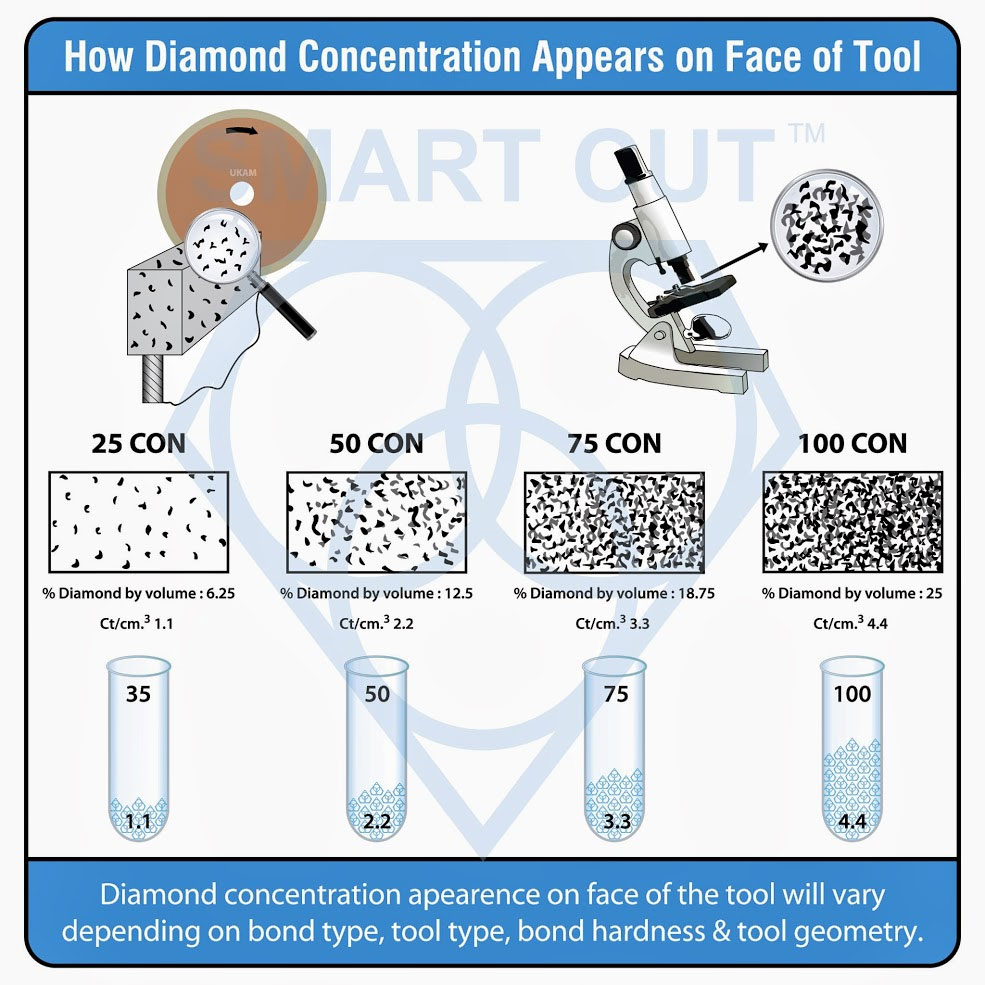
-
Improved Efficiency: High diamond concentration can distribute the cutting load more evenly, minimizing per unit force and preventing excessive deformation, particularly beneficial in metallography.
Balancing Trade-offs
The decision between high and low diamond concentrations depends largely on balancing speed versus precision and material properties versus tool cost. For instance:
-
Materials like tungsten or carbide, which are both hard and somewhat brittle, might still benefit from a medium to low diamond concentration, combining aspects of both cutting mechanics.
-
In environments where speed is less critical than cost or where material conservation is crucial, such as in gemological laboratories or delicate archaeological specimen preparation, low diamond concentration blades may be preferable despite slower cutting speeds.
Diamond Particle/Grit size
The selection of diamond mesh size is a critical factor that can significantly affect the outcome of the cutting process. Laboratory technicians must consider the specific characteristics of the material being cut, the desired outcome of the cut, and operational priorities such as speed and cost. By carefully selecting the appropriate diamond mesh size, operators can optimize the cutting process to achieve the best possible results, balancing efficiency with precision and material integrity.
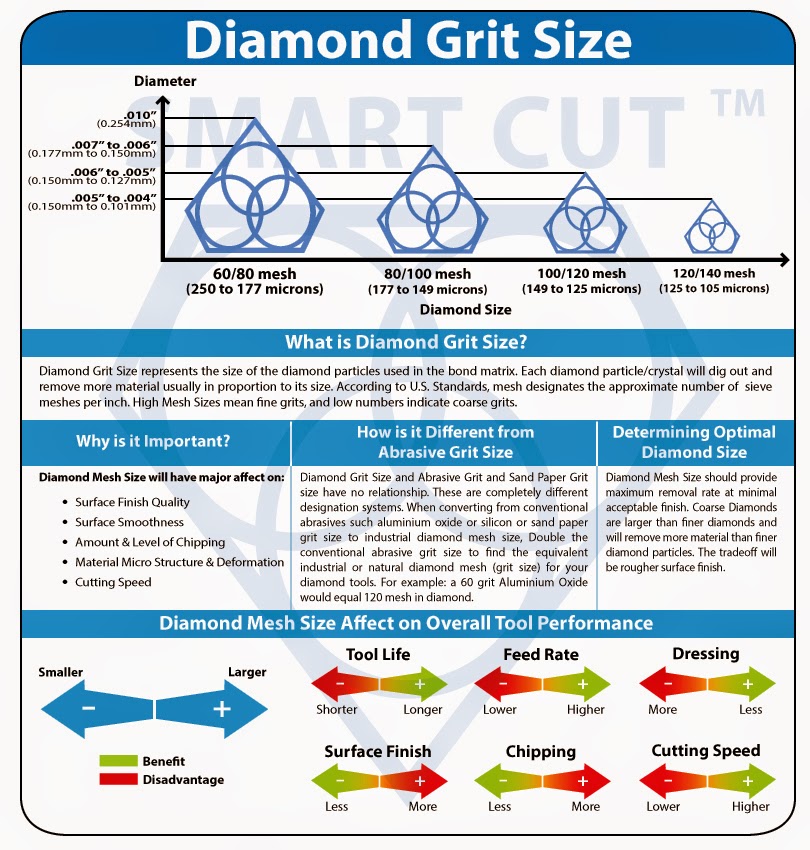
Role of Diamond Mesh Size
The diamond mesh size, or grit size, is a crucial determinant in the performance of diamond wafering blades, impacting everything from cutting speed and surface finish to the extent of chipping and potential microstructural damage to the material. Understanding how diamond mesh size influences these factors can guide users in selecting the most suitable blade for their specific applications.
Coarse vs. Fine Diamond Mesh Sizes
For cutting ultra-hard and brittle materials, such as advanced ceramics, glass, and certain composite materials, blades with a low diamond concentration are often preferred. In these blades, the distribution of diamond particles is sparser, which means that each diamond experiences more pressure during the cutting process. This high pressure facilitates a micro-fracture mechanism, where the diamonds effectively chip off small flakes from the material being cut. This method is particularly effective for brittle substrates, where the precision of the cut and the minimization of material damage are paramount.
-
Faster Cutting Speeds: Coarse diamond particles are larger, which allows them to remove more material per pass, thereby increasing the cutting speed. This can be particularly advantageous in situations where time efficiency is critical.
-
Increased Material Removal: Larger diamonds exert a greater force on the material, which can lead to increased chipping and potentially more significant microstructural damage. This might be acceptable in cases where the precision of the cut is less critical than the speed.
-
Applications: Coarse diamond grits are often preferred for robust, non-delicate materials where surface finish is not the primary concern, such as in the initial stages of shaping or bulk material removal.
Fine Diamond & CBN Mesh Sizes:
-
Improved Cut Quality and Surface Finish: Finer diamonds create smoother cuts with finer surface finishes. This is crucial for applications requiring high aesthetic quality or where further processing or analysis is needed.
-
Reduced Chipping and Microstructural Damage: The smaller size of the diamonds reduces the impact on the material’s surface, minimizing chipping and preserving the integrity of the material’s microstructure. This is particularly important for delicate or brittle materials.
-
Applications: Fine diamond grits are recommended for cutting fragile, delicate materials such as crystals, gemstones, and certain ceramics, or when preparing samples for high-precision analytical techniques.
Balancing Cutting Speed and Surface Finish / Material Deformation
Choosing between coarse and fine diamond mesh sizes involves balancing the need for quick material removal against the requirements for precision and minimal damage. For example:
-
For high-throughput industrial applications where speed is a priority, a coarser grit may be more effective.
-
In laboratory settings where precision and minimal sample damage are crucial, finer grit sizes are more appropriate.
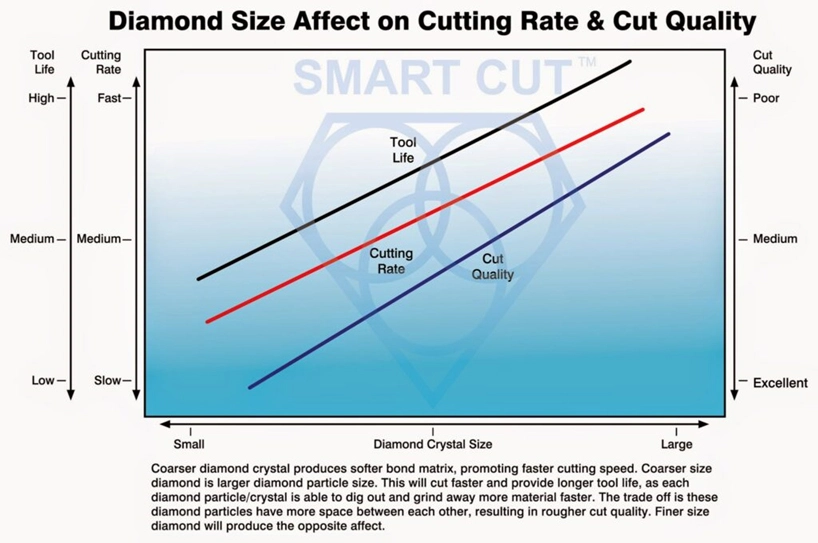
Balancing Cutting Speed and Surface Finish / Material Deformation
Choosing between coarse and fine diamond mesh sizes involves balancing the need for quick material removal against the requirements for precision and minimal damage. For example:
-
For high-throughput industrial applications where speed is a priority, a coarser grit may be more effective.
-
In laboratory settings where precision and minimal sample damage are crucial, finer grit sizes are more appropriate.
Influence on Heat Generation and Swarf Management
The size of the diamond particles not only affects the rate of material removal and the quality of the finish but also plays a significant role in the generation of heat and management of swarf (the waste material produced by cutting processes).
Coarse Diamond Mesh Sizes:
-
Increased Heat Production: The aggressive cutting action of larger diamond particles generates more friction and consequently more heat, which can be detrimental to both the blade and the material, especially those sensitive to thermal effects.
-
Swarf Size: Larger diamond particles tend to produce larger swarf, which can be easier to manage and remove from the cutting area, reducing the likelihood of clogging and maintaining effective cutting performance.
Fine Diamond Mesh Sizes:
-
Reduced Heat Generation: Finer particles create less friction and therefore less heat, preserving the microstructural integrity of heat-sensitive materials.
-
Swarf Characteristics: Fine grit sizes produce finer swarf, which can quickly accumulate and potentially clog the cutting equipment if not adequately managed.
Effect on the Diamond Bond and Blade Longevity
The mesh size also influences the choice of the bond—the material that holds the diamond particles in place on the blade. This relationship is crucial for maximizing the blade's lifespan and ensuring consistent performance throughout its use.
Coarse Diamond Mesh Sizes:
-
Bond Requirements: Coarser particles may require a stronger or more robust bond to hold the larger diamonds in place, especially under the high stress of cutting harder materials.
-
Blade Wear: While coarse grits may wear down more quickly due to their aggressive cutting nature, the right bond can mitigate premature wear and extend the blade's life.
Fine Diamond Mesh Sizes:
-
Bond Selection: Finer diamonds can be held with a less robust bond, which can be beneficial for achieving a smooth cut as the bond can wear away at a rate that exposes new, sharp diamond particles.
-
Consistent Performance: Finer grits often result in more uniform wear and a consistently high-quality finish over the life of the blade.
-
Material Hardness and Abrasiveness: The hardness and abrasiveness of the material being cut also influence the choice of diamond mesh size. Harder materials may require finer grits to achieve a quality cut without excessive tool wear.
-
Material Hardness and Abrasiveness: The hardness and abrasiveness of the material being cut also influence the choice of diamond mesh size. Harder materials may require finer grits to achieve a quality cut without excessive tool wear.
-
Cost Implications: Finer diamond particles often result in higher costs due to the additional processing required to produce smaller, consistent diamond sizes.
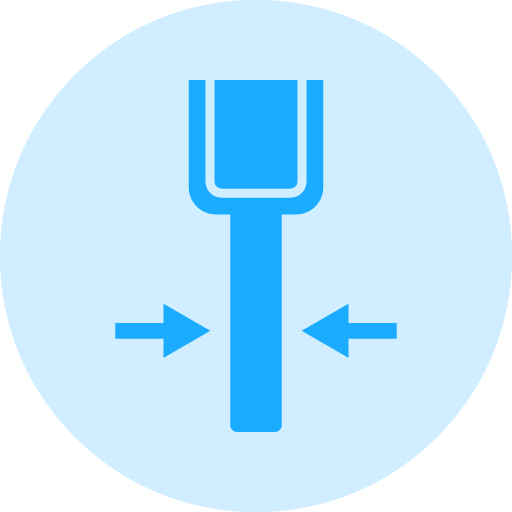
Wafering Blade Kerf Thickness
Blade thickness plays a crucial role in the efficiency and quality of sectioning materials for sample preparation. In the context of wafering blades used for precision cutting, such as in the preparation of semiconductor materials or delicate geological specimens, selecting the appropriate blade thickness is paramount to minimize material loss and ensure a precise cut.
Blade Thickness and Its Impact on Cutting Performance
Wafering blade thickness typically ranges from 0.006 inches to 0.040 inches (approximately 0.15mm to 1mm). Thinner blades, while less common, are available from stock such as 3” x .004” x ½”. This is the thinnest diamond wafering blade we have in stock. Other kerf thicknesses may be produced upon request.
The kerf—defined as the width of material removed during the cutting process—generally increases with the blade diameter. This proportionate increase is critical as it affects the amount of material lost during sectioning, which is a significant consideration for applications requiring high material conservation, such as in costly or rare sample preparations.
For applications necessitating high-precision positioning of the cutting plane—such as in integrated circuits or other microscale devices—a thinner, smaller-diameter blade is preferable. Blades ranging from 3 inches to 5 inches (75mm to 125mm) in diameter and thicknesses from 0.006 inches to 0.015 inches (0.15mm to 0.4mm) are typically suited for such purposes. These dimensions allow for enhanced precision without excessive material waste, crucial in maintaining the integrity of microscale features.
Factors Influencing the Choice of Blade Thickness
The optimal blade thickness for a given material and application involves a consideration of multiple factors:
-
Cutting Speed: Thinner blades can enable faster cutting speeds, beneficial for operations requiring quick turnaround times.
-
Load/Feed Rate: The load that can be sustained by the blade without compromising its integrity or the quality of the cut depends significantly on the blade's thickness. Thicker blades can withstand higher loads and feed rates.
-
Material Properties: The hardness, density, and overall size of the material being cut are crucial in determining the suitable blade thickness. Harder materials generally require thicker blades to prevent blade wear and ensure clean cuts.
-
Operator Skill and Experience: Thicker kerf blades offer more forgiveness for operator error and are less likely to break under less-than-ideal operating conditions. This makes them suitable for environments with operators of varying skill levels, such as educational settings or shared facilities.
Recommendations for Various Environments
In settings where the equipment is used by a diverse group of individuals, such as university laboratories or communal workshops, thicker kerf blades are advisable. Their robustness and tolerance for higher loads and potential operator mishandling make them ideal for novice users or when frequent blade changes are impractical.
Conversely, in laboratory or industrial environments where the blades will be used on precision computer controlled machines and experienced operators, and where minimizing material loss and maximizing accuracy are paramount, selecting a thinner blade is essential. Such blades are more suitable for experienced operators who can handle the equipment with the necessary care and expertise to exploit the advantages of a thinner kerf.
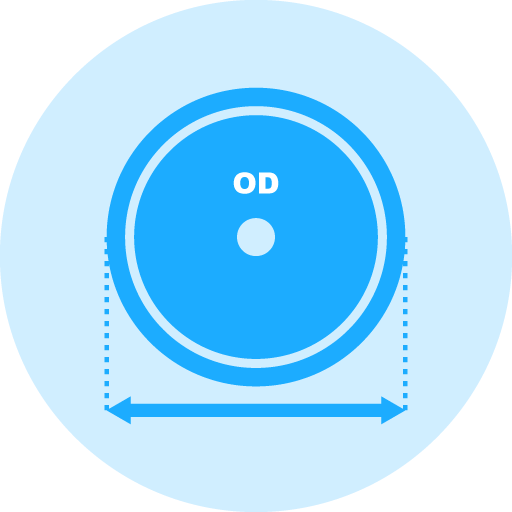
Wafering Blade Outside Diameter
The diameter of a wafering blade is a fundamental factor that affects the cutting performance, blade stability, and overall effectiveness of the cutting process. Understanding the implications of blade diameter can help users choose the most appropriate blade for their specific material and application needs.
Range and Implications of Blade Diameters
Wafering blades typically come in diameters ranging from 3 inches (75mm) to 8 inches (200mm). The choice of diameter is closely linked to the dimensions and physical characteristics of the material being cut.
Smaller Diameter Wafering Blades:
-
Precision in Smaller Samples: Smaller blades are generally used for precise cutting of smaller diameter samples on both low and high speed sectioning / wafering saws. Their reduced size allows for finer control and minimal material loss, which is crucial in applications such as sample preparation, metallography and material research.
-
Potential for Flexibility and Distortion: Due to their thinner profile, smaller diameter blades can be more prone to bending and warping under pressure. This requires careful handling and operation, especially at higher speeds or when making deep cuts in hard materials.
Larger Diameter Wafering Blades:
-
Suitability for Larger, Heavier Samples: Larger blades are capable of handling bigger and heavier materials, making them ideal for industrial applications or when cutting thick, dense substances.
-
Increased Stability and Durability: The thicker profile of larger diameter blades provides greater stability, reducing the likelihood of blade deflection and ensuring more consistent cuts. This robust construction allows them to withstand higher loads and operate at higher speeds without significant degradation.
Factors Influencing the Selection of Blade Diameter
-
Sample / Material Size: The diameter and thickness of the material being cut are perhaps the most critical factors. A blade diameter should be chosen such that it allows the blade to cut through the material without excessive overhang, which can lead to blade instability and uneven cutting.
-
Cutting Depth and Reach: Larger blades can reach deeper into the material, which is beneficial for sectioning thick or layered materials. They also maintain better angular accuracy due to their increased rigidity.
-
Operational Speeds: Larger blades can typically operate at higher speeds because of their increased stability and heat dispersion capabilities. This makes them suitable for applications requiring quick throughput.
-
Machine Compatibility: The choice of blade diameter must also consider the specifications and limitations of the cutting equipment being used. Compatibility with the machine’s range of operation, power output, and safety features is essential for effective cutting.
Impact of Blade Diameter on Load Distribution and Heat Management
The diameter of the blade not only influences the stability and potential for material handling but also plays a crucial role in how the blade distributes mechanical stress and manages thermal buildup during the cutting process.
Larger Diameter Wafering Blades:
-
Enhanced Load Distribution: The increased surface area of larger blades allows for a more even distribution of forces exerted during cutting. This reduces the stress on any single point of the blade, enhancing its durability and reducing the risk of breakage.
-
Improved Heat Dissipation: Larger blades generally have a greater mass and surface area, which help in dissipating heat more effectively. This is particularly important when cutting materials that are sensitive to heat or during prolonged cutting operations which can generate significant heat.
Smaller Diameter Wafering Blades:
-
Precision Handling: While smaller blades can heat up more quickly due to their limited surface area, their size allows for greater precision and control, especially in intricate cutting tasks where minimal deviation is critical.
-
Adaptability to Intricate Cuts: Their ability to maneuver in tight spaces makes them ideal for applications requiring detailed shapes or patterns, such as in the creation of micro components or detailed gem cutting.
Strategic Use of Blade Diameter Based on Cutting Mechanics
The mechanics of cutting—whether by abrasive action, chipping, or a combination of both—can also influence the optimal blade diameter for specific materials and cuts.
-
Abrasive Materials: When cutting highly abrasive materials, larger blades can provide the robustness needed to withstand wear and tear while maintaining cutting effectiveness.
-
Brittle Materials: Conversely, smaller blades are typically more effective for brittle materials, as they can make precise cuts with minimal pressure, reducing the risk of cracking or breaking the material.
Improving Blade Life and Overall Performance
Maintaining optimal blade life and performance involves more than selecting the right diameter; it also includes regular maintenance and proper usage.
-
Blade Alignment and Calibration: Regular checks for blade alignment and calibration are vital, especially for smaller diameter blades that are more susceptible to deviations which can affect cut quality and blade life.
-
Appropriate Speed Settings: Matching the blade speed to the diameter is essential for maximizing performance and safety. Smaller blades often require higher speeds to achieve effective cutting, whereas larger blades may perform better at lower speeds due to their increased mass and cutting power.
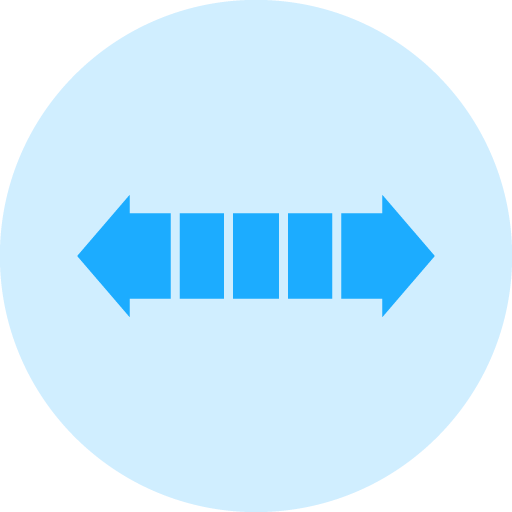
Bond Hardness
Choosing the right bond hardness is essential for optimizing the performance and lifespan of a wafering blade. By understanding the interactions between the wafering blade's bond hardness, the material being cut, and the desired goal, users can make informed decisions that enhance the efficiency and effectiveness of their sectioning operations. This strategic selection ensures that the sectioning operation provides maximum performance, balancing wear resistance, cutting speed, and overall blade life.
Understanding Bond Hardness
Bond hardness in diamond & cbn wafering blades refers to the capacity of the bond matrix to securely hold diamond particles. This attribute is critical because it directly affects the blade's performance, including its cutting speed, life expectancy, and the quality of the cut. The bond matrix's hardness is typically gauged on a scale ranging from soft to hard, with numerous intermediate variations designed to match specific cutting requirements and material characteristics.
Balancing Diamond Retention and Cutting Speed
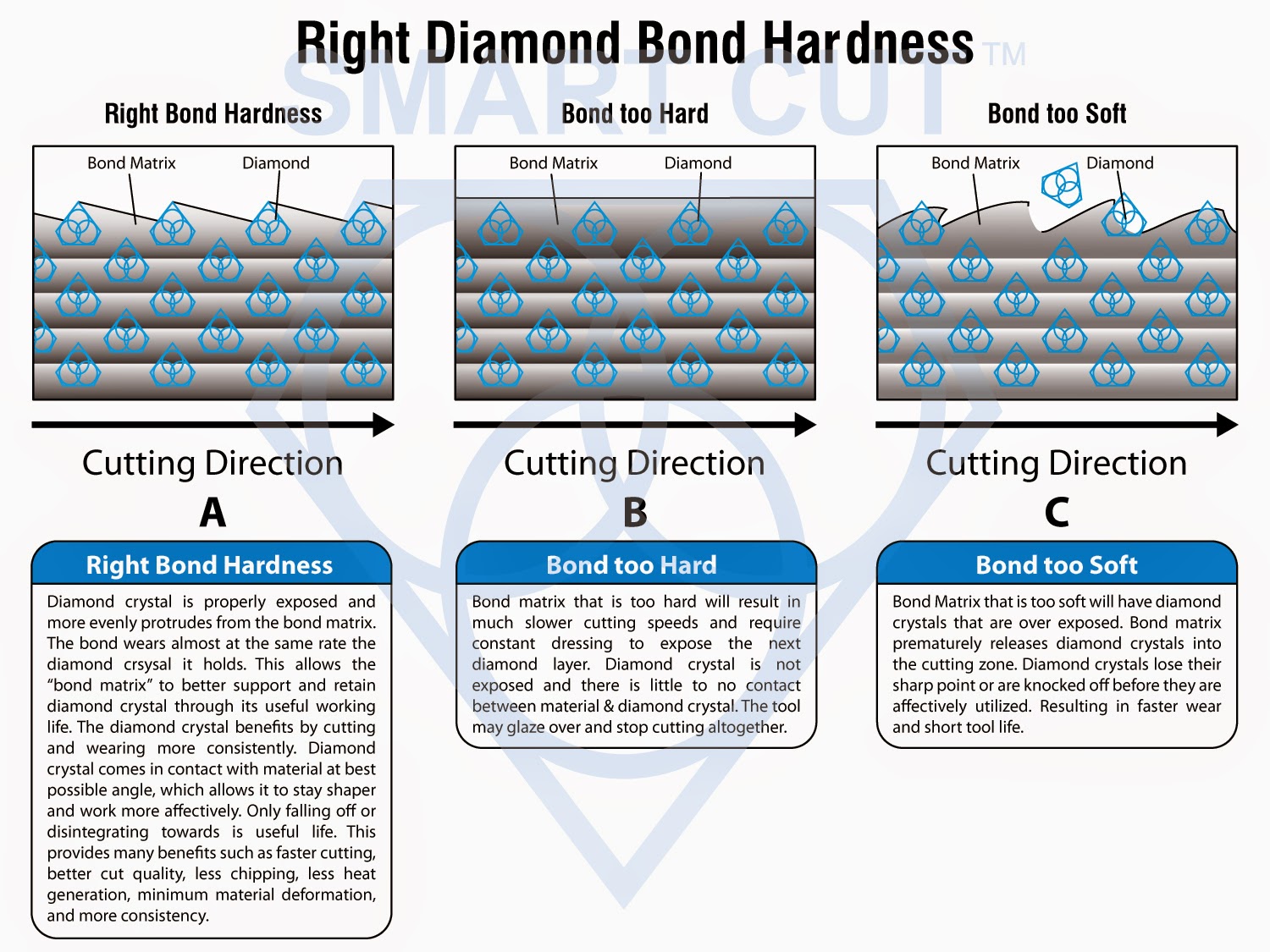
Increased Bond Hardness:
-
Enhanced Diamond Retention: Harder bonds are better at holding onto diamond particles, which is beneficial for prolonging the blade's operational life. This characteristic is particularly valuable when cutting abrasive materials that could otherwise rapidly wear down the bond.
-
Trade-off in Cutting Speed: While harder bonds excel in retention, they generally result in slower cutting speeds. The diamonds are not exposed as quickly or as frequently, requiring more time to cut through material.
-
Need for Dressing: Blades with harder bonds often require more frequent dressing to expose fresh diamond particles. This is necessary to maintain effective cutting capability and to prevent glazing of the blade surface.
Decreased Bond Hardness:
-
Faster Cutting Speed: Softer bonds tend to release diamond particles at a faster rate, allowing for new, sharp diamonds to come into contact with the material more quickly. This leads to faster cutting speeds but can increase the rate at which the blade wears.
-
Reduced Blade Life: A softer bond generally means that the blade will wear out faster, as the diamonds are released more quickly and the bond itself is abraded away.
Optimizing Bond Hardness for Material Types
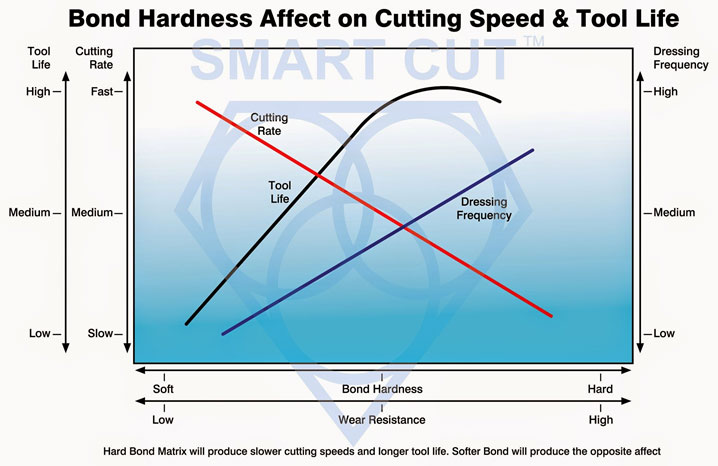
-
Harder Materials: Materials like sapphire, quartz, and alumina typically require a blade with a softer bond. The softer bond allows for the quicker release of diamonds, which is necessary to cut through these hard materials without excessive force, thereby reducing the risk of cracking or damaging the material.
-
Softer and More Brittle Materials: Softer materials such as calcareous stones or certain ceramics often benefit from a harder bond. The increased retention of diamonds ensures that the blade can maintain its cutting effectiveness over a longer period, which is crucial when handling materials that might otherwise cause rapid bond erosion.
Bond Hardness Classification
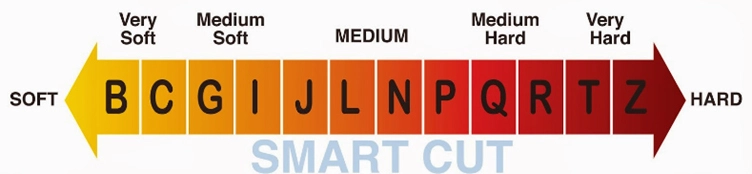
The diamond and cbn wafering blades have different classification schemes to categorize bond hardness, often based on the specific composition of the bond matrix and its performance characteristics. These classifications help users select the most appropriate blade for their particular application. Factors considered in these classifications include:
-
Abrasive Resistance: The ability of the bond to resist wear from abrasive materials.
-
Thermal Stability: How well the bond can maintain its properties under the heat generated by cutting.
-
Mechanical Strength: The overall durability of the bond under mechanical stresses.
Wafering Blade Bond Hardness Considerations
When selecting a wafering blade, it is crucial to consider the specific needs of the application:
-
Material to be Cut: Assess the hardness and abrasiveness of the material.
-
Desired Cut Quality and Speed: Determine if the priority is on achieving high-quality cuts or faster completion.
-
Equipment Used: Ensure that the wafering blade is compatible with the cutting equipment in terms of size, power, and operational speed.
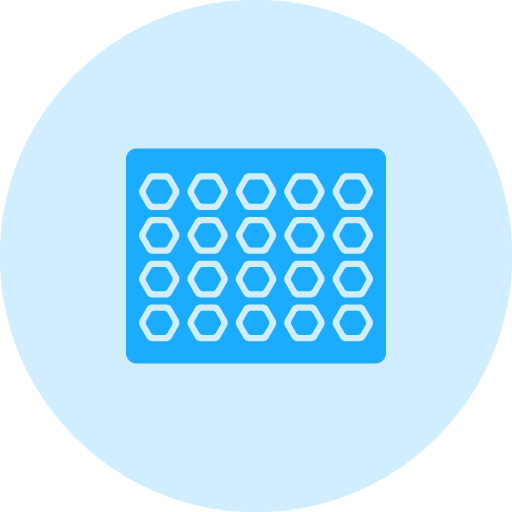
Bond Type for Diamond & CBN Wafering Blades
The bond type in wafering blades is a critical factor that determines their suitability for various applications, directly affecting the wafering blade's life, the heat generated during cutting, and the quality of the finish. The of the most common types of bonds are metal resin, and nickel bond. Each offering distinct advantages and specialized uses in metallography, sample preparation and materials research application
Sintered (Metal Bond) Diamond Wafering Blades
About Sintered (Metal Bond)
Sintered (Metal Bond) diamond & CBN wafering blade have multiple layers of diamonds impregnated inside the metal matrix. Diamonds are furnaces sintered in a matrix made of iron, cobalt, nickel, bronze, copper, tungsten, alloys of these powders or other metals in various combinations. Metal bonded wafering blades are “impregnated” with diamonds. This means that selected diamonds are mixed and sintered with specific metal alloys to achieve the best cutting performance possible on any materials such as sapphire, advanced ceramics, optics, glass, granite, metals and etc. The metal bond surrounding the diamonds must wear away to continuously keep re-exposing the diamonds for the diamond tool to continue cutting. Sintered (metal bonded) diamond wafering blades are recommended for machining hard materials from 45 to 75 on rockwell scale (4 to 9.5 on mohe’s scale of hardness).
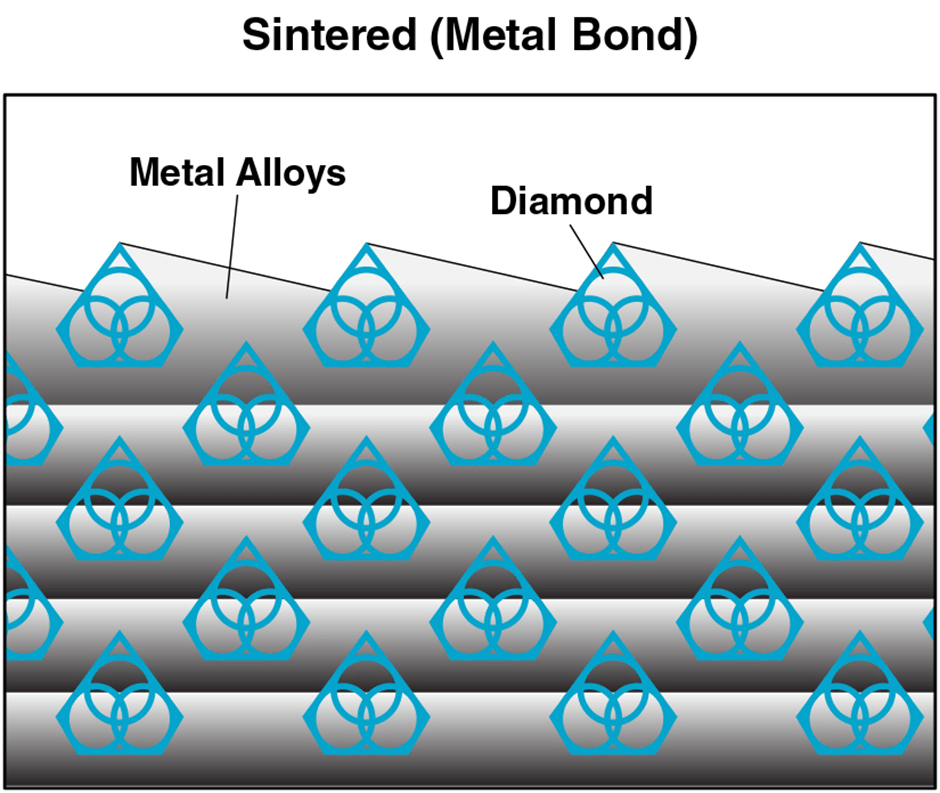
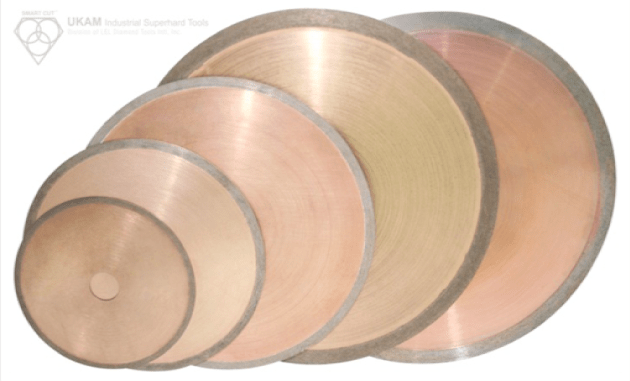
Characteristics:
-
Durability and Longevity: Metal bonds are composed of various metals or alloys, which can include bronze, brass, or even steel and nickel. This composition gives the metal-bonded diamond blades a robust structure, allowing them to maintain their integrity under harsh cutting conditions and over prolonged use.
-
Excellent Retention: Metal bonds typically have excellent diamond retention, which reduces the rate at which diamonds are lost from the blade. This characteristic is particularly beneficial when cutting very hard materials, where premature diamond loss can significantly affect performance.
-
Cutting Ability: These blades are well-suited for cutting hard, dense materials such as stone, concrete, and hardened metals. Their strength allows them to endure the stresses involved in cutting these tough materials without excessive wear.
Limitations:
-
Heat Generation: Metal bonds tend to generate more heat during cutting compared to other bond types. This can be a disadvantage when working with heat-sensitive materials as it might lead to thermal damage or structural changes in the material being cut.
-
Surface Finish: While metal-bonded blades are highly durable, they may not always provide the smoothest finish, potentially leaving marks or rough surfaces that might require additional finishing processes.
-
Extended Use Scenarios: Metal bonded blades are particularly well-suited for continuous, heavy-duty applications where blade longevity and durability are paramount. They are often the choice for large-scale construction projects, mining operations, and in the processing of natural stones.
-
Customizable Metal Bonds: Advances in metallurgical technologies allow for the customization of metal bonds according to specific performance requirements. Manufacturers can alter the metal matrix to balance between wear resistance and diamond exposure, tailoring blades for optimal performance in targeted applications.
Technical Enhancements:
-
Improved Heat Management: Techniques such as improved cooling systems and blade geometry optimizations are being developed to mitigate the heat generation issues commonly associated with metal bonds. These innovations enhance the blade's performance, especially in heat-sensitive cutting environments.
-
Softer and More Brittle Materials: Softer materials such as calcareous stones or certain ceramics often benefit from a harder bond. The increased retention of diamonds ensures that the blade can maintain its cutting effectiveness over a longer period, which is crucial when handling materials that might otherwise cause rapid bond erosion.
About Resin Bond
Resin bond wafering blades are made with high temperature phenolic resin, diamond particles, ceramic filler and often additional additives. Resin Bond is the softest of all the bonds, frequently used in applications that require a smooth surface. This type of wafering blade is used on majority of ultra hard & brittle materials. Resin bond wafering blades allow the bond matrix to wear faster, and allow for diamond particles/crystals to break out of their matrix faster, so that new sharp diamond particles become exposed. This self sharpening characteristic of resin bond wafering blades, based on faster bond wear out is what make a good choice where excellent surface finish cut quality is essential. Typical Resin Bond wafering Blades last less than Sintered (Metal Bond) diamond wafering blades, but more than electroplated (nickel bond) diamond blades.
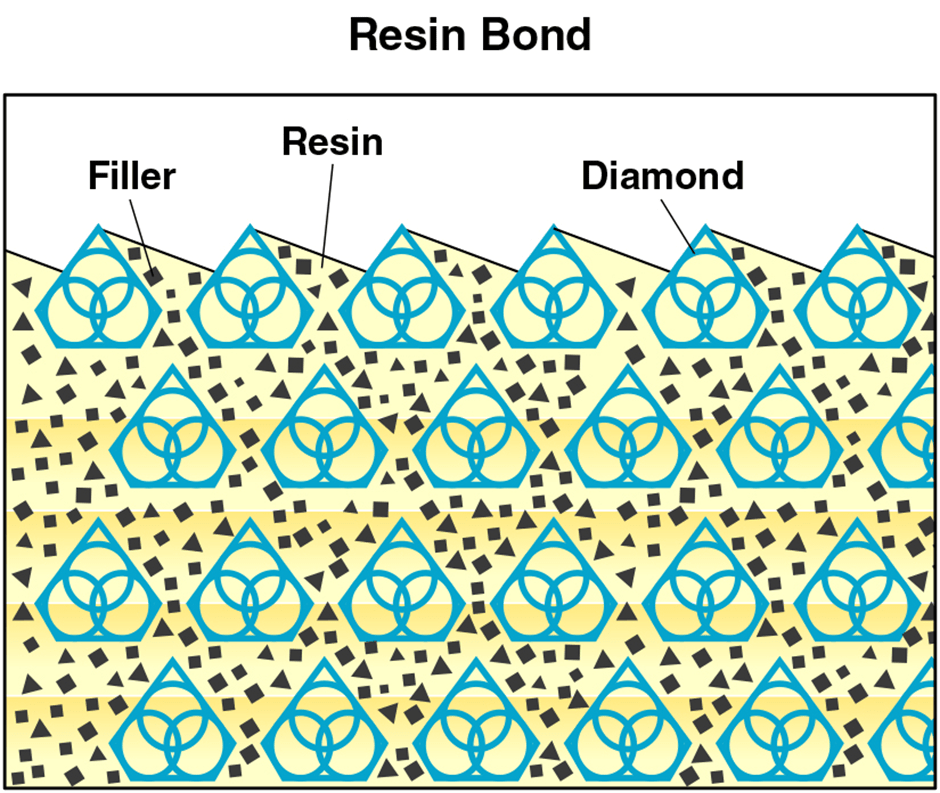
Resin Bonded Diamond Blades Characteristics:
-
Reduced Heat Production: Resin bonds typically generate less heat during cutting, making them ideal for materials that are sensitive to high temperatures. This feature helps preserve the material’s integrity and maintains its properties post-cutting.
-
Smooth Surface Finish: Resin-bonded blades are known for providing excellent surface finishes. This makes them suitable for applications where a smooth, clean cut is crucial, such as in the cutting of semiconductors, gemstones, or fine ceramics.
-
Versatility: They are particularly effective for cutting hard, delicate, or brittle materials. The softer nature of the resin bond allows for a smoother cutting action, minimizing the risk of chipping or cracking the material.
Limitations:
-
Wear Rate: Resin bonds tend to wear down faster than metal bonds, especially when used on very hard or abrasive materials. This higher wear rate can lead to increased costs and downtime due to more frequent blade replacements.
-
Structural Integrity: Under heavy load or in aggressive cutting environments, resin-bonded blades might not maintain their structural integrity as effectively as metal-bonded blades, leading to potential blade deformation or breakage.
About Nickel Bond (Electroplated) Diamond Wafering Baldes
Electroplated (nickel bond) diamond or cbn wafering usually have a single layer of diamonds, held by a tough durable nickel alloy. Nickel is frequently used as a base for plating diamond. Because of its excellent strength, toughness and flexibility during the plating process. Electroplated wafering blades are able to retain their original shape and dimensions thought their working life. Unlike sintered (meal bond) or resin bond diamond wafering blades, where diamond particles are buried in bond and held together by metal or resin binder deep inside. Electroplating allows diamond particles to protrude from the bond matrix, providing a free, faster cutting action with minimum heat generation.
Recommended for cutting softer and more gummy materials, where sintered (metal bond) diamond wafering blades load up or glaze over frequently. Unlike sintered (meal bond) cutting blades where diamond is impregnated inside metal binder matrix based on bronze, copper, nickel and other alloys. Nickle bond blades have diamond exposed right on surface of the blade. Hence they are able to provide more faster and freer cutting action than their sintered (metal bond) counterparts.
Nickel bond diamond wafering blades are particularly well suited for cutting thermosetting plastics, GRP, pre-sintered and pre-fired (green) materials, electro carbons, graphite, soft ferrites, farinaceous products, deep frozen fish, pones, pc boards, and etc.
Characteristics of Nickel Bond/Electroplated Diamond Wafering Blades
High Precision:
Electroplated diamond wafering blades are engineered for accuracy. The fine layer of diamond particles allows for extremely precise cuts with minimal material loss, making them ideal for intricate sectioning and thin slicing.
Durability:
The electroplated bond between the diamond particles and the metal substrate ensures high durability and resistance to wear, even when cutting hard or abrasive materials.
Low Kerf:
These blades are generally thin, leading to a narrow kerf (cut width). This feature is beneficial for reducing material waste and ensuring clean cuts with minimal deformation.
Versatility:
Electroplated blades can cut a variety of materials, from ceramics and glass to composites and metals. This versatility makes them useful in numerous industries, including manufacturing, research, and electronics.
Reduced Heat Generation:
Due to their thin profile and high precision, these blades generate less heat during cutting, reducing the risk of thermal damage to sensitive materials.
Ease of Use:
These blades are typically easy to install and maintain. They can be used with various precision cutting machines, such as tabletop saws and precision wafering devices.
Clean Cutting Surface:
The smooth and precise cuts from these blades lead to cleaner surfaces, requiring less post-processing and reducing the need for additional finishing operations.
Cost:
Electroplated diamond blades tend to be less costly than other types of wafering blades, reflecting their precision and durability.
Limitations of Electroplated Diamond Wafering Blades
Limited Cutting Depth:
Due to their thin profile, these blades may have a limited cutting depth, which can restrict their use in applications requiring deeper cuts.
Reduced Flexibility:
Electroplated blades are relatively rigid, which can be a limitation when cutting complex shapes or curved lines. They are best suited for straight or minimally curved cuts.
Limited Lifespan:
Although durable, the electroplated bond may eventually wear down, causing the diamond particles to detach from the metal substrate. This can lead to reduced cutting efficiency and a need for replacement.
Sensitive to Improper Use:
These blades are designed for specific applications, and improper use (such as applying excessive force or cutting inappropriate materials) can lead to damage or accelerated wear.
Limited Reusability:
Once the diamond coating wears off, these blades cannot be reconditioned like other types of blades, such as resin-bonded or metal-bonded diamond blades.
Choosing the Right Bond Type
The choice between metal, resin, nickel bond wafering blades should be based on a thorough assessment of the material to be cut, the desired objective, the specific cutting conditions, and the equipment used. Here are some considerations to guide the selection process:
-
Material Hardness and Abrasiveness: For harder and more abrasive materials, metal bonds are generally preferred due to their durability and longevity. For materials that are hard but brittle or delicate, resin bonds are often more suitable due to their ability to cut cleanly without inducing stress fractures.
-
Finish Requirements: If the priority is achieving a high-quality finish with minimal post-processing, resin-bonded blades are the better choice.
-
Heat Sensitivity: For materials sensitive to heat, such as thermoplastics or certain metals that might temper under heat, using a resin-bonded blade is advisable.
Diamond Depth / Height
The depth or height of the diamond section indicates how much diamond material is available for cutting. Greater diamond depth typically leads to longer blade life because there's more material to work with before the blade becomes ineffective. A deeper diamond section can also mean less frequent maintenance and fewer replacements.
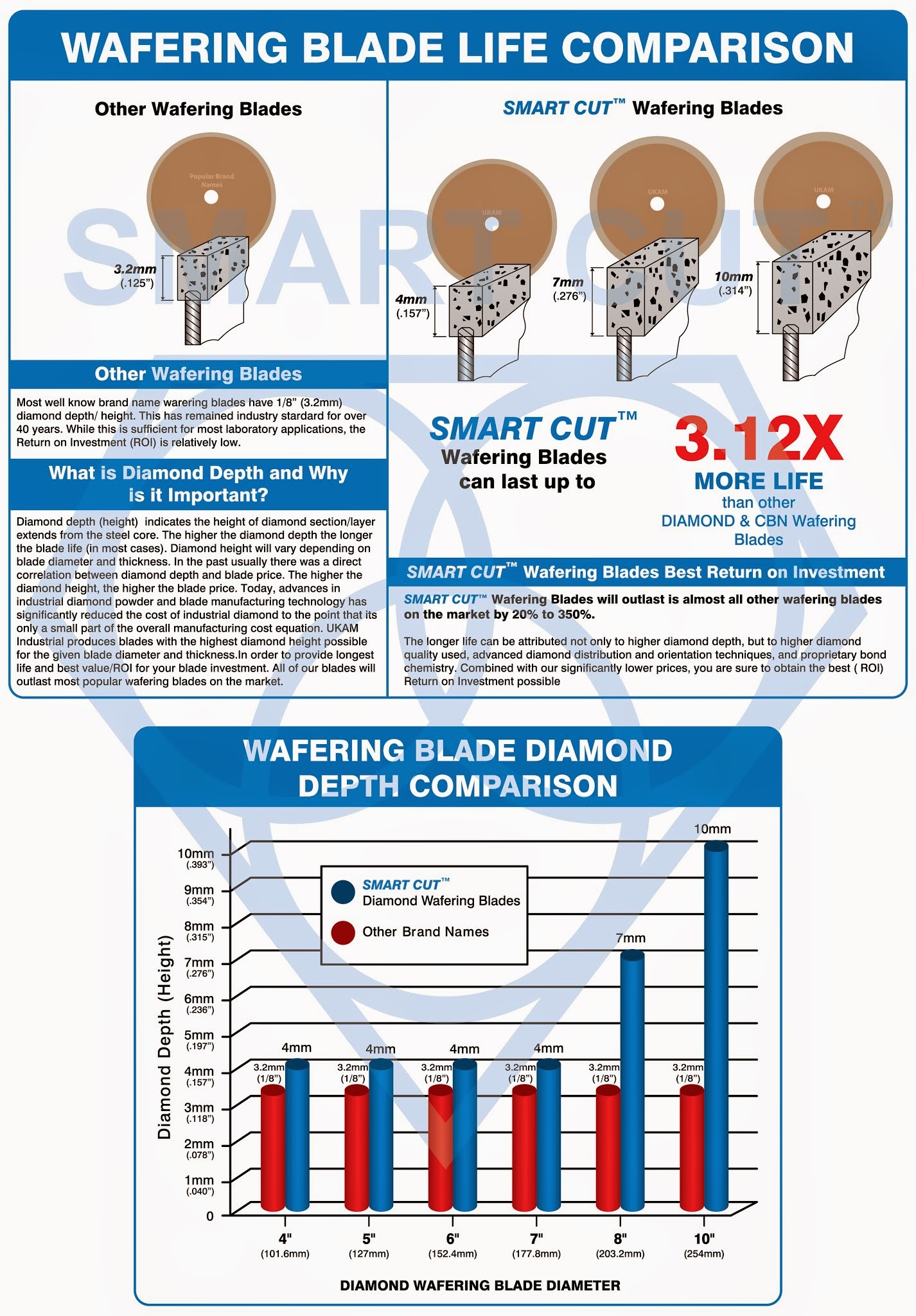
Comparison of Wafering Blade Durability
Traditional Wafering Blades vs. SMART CUT™ Wafering Blades
Traditional wafering blades in the industry often come with a standard diamond depth of 1/8" (3.2mm), a norm that has been largely unchanged for the past 40 years. While this depth serves most laboratory applications adequately, the Return on Investment (ROI) can be considerably low.
The Significance of Diamond Depth
Diamond depth refers to the thickness of the diamond-containing segment or layer on the blade, extending from the blade's core. The greater the diamond depth, the longer the blade's lifespan since the wear rate is distributed over a larger volume of diamond. Historically, there was a clear correlation between diamond depth and blade pricing. With advancements in industrial diamond tool manufacturing technology, it's now possible to provide blades with deeper diamond layers at cost-effective pricing. UKAM Industrial Superhard Tools has innovated in this area, delivering blades that offer the maximum depth for diamonds without inflating costs. This ensures that our customers receive the most efficient and cost-effective blade, with a lifespan that outperforms the most popular blades on the market.
SMART CUT™ Wafering Blades: Enhanced Longevity and Value
SMART CUT® Wafering Blades can offer up to 3 to 12 times the lifespan compared to other diamond and CBN wafering blades, offering the best return on investment. They outlast competitors' blades by 20% to 35%, attributable not only to greater diamond depth but also to superior diamond quality, advanced distribution, and proprietary bonding chemistries. With significantly competitive pricing, SMART CUT® blades ensure customers get the best ROI possible.
Diamond Wafering Blade Diameter vs. Diamond Depth Analysis
When comparing the diamond depth across various blade diameters, SMART CUT® shows a consistent diamond depth, significantly surpassing that of other brands. As the blade diameter increases from 4 inches (101.6mm) to 10 inches (254mm), SMART CUT® maintains a diamond depth that offers greater durability and cutting performance.
-
For blade diameters of 4", 5", 6", 7", and 8", SMART CUT™ and other brands offer a diamond depth of 3.2mm.
-
At a 10" blade diameter, SMART CUT™ provides a remarkable 10mm diamond depth, in contrast to other brands that typically offer only 7mm.
This depth consistency across different blade sizes ensures that regardless of the blade diameter, users experience superior cutting action and extended blade life with SMART CUT® .
Superior Durability with Advanced Diamond Depth
The SMART CUT® Wafering Blades set a new industry benchmark with their exceptional diamond depth. Unlike traditional blades that adhere to the 1/8" (3.2mm) standard diamond depth, SMART CUT® has revolutionized the norm by offering up to a remarkable 10mm depth for larger diameter blades. This innovation translates to a significant extension in blade life—by as much as 3 to 12 times—compared to standard diamond and CBN wafering blades.
Optimized Return on Investment with SMART CUT™
Investing in SMART CUT™ Wafering Blades ensures a maximized Return on Investment (ROI). These blades have been engineered to surpass the longevity of other blades on the market by 20% to 35%, owing to the deeper diamond layer and the utilization of premium-quality diamonds. The advanced distribution technology and proprietary bond chemistry of SMART CUT™ also contribute to its prolonged life and superior performance. These factors, combined with competitive pricing, mean that customers can achieve the best possible ROI, gaining both in terms of blade life and cost savings over time.
Consistency Across Diameters in SMART CUT™ Blades
SMART CUT™ maintains a consistent diamond depth regardless of blade diameter, ensuring that users experience uniform cutting quality and longevity across all blade sizes. While both SMART CUT™ and other brands start with the same diamond depth at smaller diameters (3.2mm), SMART CUT™ continues to provide this depth even as the blade diameter increases. At a substantial 10-inch diameter, the SMART CUT™ blade boasts a diamond depth of 10mm, a considerable increase over the typical 7mm provided by other brands.
Advanced Manufacturing for Peak Performance
SMART CUT™ employs the latest in diamond tool manufacturing technology to ensure that each blade is not only robust but also performs at peak efficiency. The higher diamond depth, reduces the need for frequent blade changes, thus maintaining continuity in precision work and reducing downtime. The robust design is especially beneficial for industries where the cutting of hard, abrasive materials is common, ensuring that the blade's performance does not diminish over extended use.
Tailored for a Variety of Materials and Applications
The SMART CUT™ blade range is designed to tackle a diverse array of materials, from the softest polymers to the hardest ceramics. By maintaining a uniform diamond depth across various diameters, SMART CUT™ blades provide the flexibility to handle different materials and applications without compromising on quality or efficiency. This versatility makes SMART CUT™ blades ideal for specialized applications, including precision sample preparation in scientific research, semiconductor manufacturing, and fine craftsmanship where precision and minimal material loss are critical.
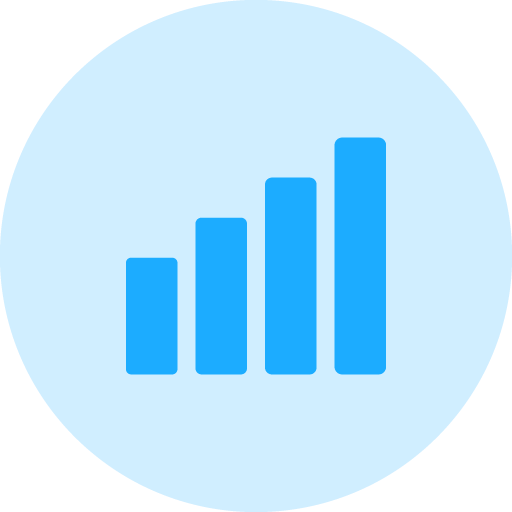
Feed Rates
Selecting the appropriate feed rate for wafering blades is a nuanced process that hinges on understanding the material's properties, the cutting equipment's capabilities, and the overall goals of the specimen preparation. By carefully balancing these factors, operators can maximize both the efficiency of their cutting processes and the quality of the resulting sections, ensuring that the specimens are cut to precise standards suitable for their intended applications. In an industry where precision is paramount, mastering the art of feed rate adjustment is key to achieving repeatable and reliable cutting outcomes.
Understanding Feed Rates in Cutting Operations
Feed rates, the speed at which a specimen is fed into a wafering blade, are a crucial parameter in precision cutting. They typically range from a light touch of 10 grams to a substantial force of 1000 grams. This range accommodates the wide variety of materials that might be encountered in industrial and laboratory settings, each requiring a customized approach based on its properties and the desired outcome of the cutting process.
Tailoring Feed Rates to Material Hardness
For Harder Specimens:
-
Optimized Load and Speed: Hard materials like ceramics and minerals necessitate higher loads and speeds during cutting. This is because such materials require a greater force for the diamonds in the blade to penetrate and section the specimen effectively. Adjusting the feed rate to match the specimen’s hardness ensures that the blade cuts efficiently without causing undue stress on the equipment or excessive wear on the blade.
-
Consistency in Cutting: Higher loads and speeds must be applied consistently to ensure uniform cuts. Any fluctuation can affect the quality of the sections and potentially damage the material being cut.
For More Brittle Specimens:
-
Gentler Approach: Brittle materials, such as silicon substrates used in electronics, require a gentler approach with lower loads and speeds. This careful handling is essential to prevent cracking or chipping, which can ruin the integrity of the specimen and potentially invalidate any subsequent analysis or processing.
-
Precision Over Speed: When cutting brittle specimens, the emphasis is on precision rather than speed. The feed rate is carefully controlled to ensure that each cut is as accurate as possible, even if this means a slower overall cutting process.
Equipment Speed and Specimen Shape:
-
Speeds/RPMs:The rotational speed of the blade, measured in RPMs (revolutions per minute), directly influences the effectiveness of the cutting action. Higher speeds can sometimes compensate for a lower feed rate, and vice versa.
-
Specimen Geometry: The shape and size of the specimen can dictate how it interacts with the blade. Irregular shapes might require slower feed rates to allow for a smooth cutting action without binding or causing errors in the cut.
Clamping and Holding Techniques:
-
Clamping Stability: The method by which the specimen is clamped or held in place during cutting plays a significant role in determining the viable load. A securely clamped specimen can typically withstand a higher feed rate, whereas a specimen that is not well-secured may require a more cautious approach.
-
Cutting Environment: The presence of coolants, the type of holding fixtures, and even the ambient temperature of the cutting environment can impact the feed rate, necessitating adjustments to achieve optimal cutting conditions.
Critical Analysis of Feed Rate Variables
Feed rates are not just numerical values to be input into a machine; they are crucial variables that can be fine-tuned to achieve the perfect balance between cut quality and efficiency. They must be determined with a comprehensive understanding of the material's response to cutting forces and the dynamic behavior of the wafering blade under varying loads.
Material-specific Feed Rate Strategies
Managing Hard Materials:
-
Load Calibration: For hard materials, the feed rate is calibrated to apply enough load to penetrate the specimen without overburdening the blade. The load must be sufficient to enable the diamond edges to engage the material effectively, promoting efficient cutting without causing thermal or mechanical overload.
-
Speed Synchronization: The synchronization of blade speed with feed rate is key. A higher RPM may allow for a proportionally higher feed rate, enabling the blade to cut through hard materials smoothly and reduce sectioning time.
Preserving Brittle Materials:
-
Minimized Mechanical Stress: Lower feed rates are essential to minimize mechanical stress when cutting brittle materials. The application of load must be carefully controlled to prevent the propagation of microcracks which could compromise the integrity of the specimen.
-
Gradual Engagement: A gradual increase in feed rate might be employed at the onset of the cutting process to prevent shock loading, followed by a steady feed once the blade has established a cutting groove.
Integration of Cutting Dynamics
Blade Speed and Feed Rate Interplay
-
Optimized Dynamics: The relationship between blade speed (RPMs) and feed rate (grams) is dynamic and integral to the cutting process. Operators must find an optimal interplay between these two factors to ensure the smoothest cut with the least amount of material deformation or blade wear.
Specimen Geometry Considerations:
-
Complex Shapes: For specimens with complex geometries, feed rates may need to be varied during the cutting process. Areas with increased cross-sectional density might require slower rates, whereas thinner sections can be cut more quickly.
Secure Clamping for Consistent Feed Rates
Clamping Efficiency:
-
Secure and Stable: Efficient clamping mechanisms are crucial for consistent feed rates. A specimen that is securely fixed in place can endure a more aggressive feed rate, which can significantly reduce cutting times.
-
Adaptive Clamping Systems: Adaptive clamping systems that can accommodate varying specimen shapes and sizes enable operators to maintain consistent feed rates across a range of cutting scenarios, ensuring the stability of the specimen throughout the cutting process.
Feed Rate Adaptability and Machine Automation
Intelligent Cutting Systems:
-
Feedback Loops: Modern sectioning saws come equipped with sensors and feedback systems that can adjust feed rates in real-time based on the resistance encountered during cutting, thereby optimizing the process for speed and quality.
-
Programmable Settings: Automated systems allow for the programming of specific feed rate profiles tailored to the specimen material and desired outcome, streamlining the cutting process and enhancing repeatability.
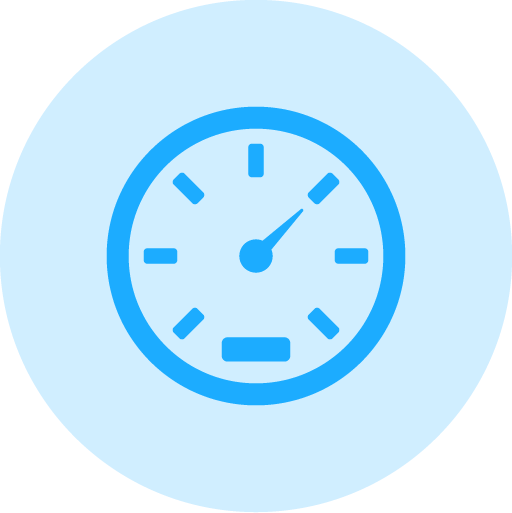
Blade Speeds/RPM’s
Wafering Blade speeds play a critical role in the sectioning process, impacting everything from the rate of material removal to the quality of the final section. By selecting the appropriate RPMs based on material properties and cutting equipment capabilities, operators can ensure a balance between cutting efficiency and sample integrity.
The Role of Blade RPM in Material Sectioning
Wafering Blade speed, measured in revolutions per minute (RPM), is a pivotal factor that directly influences the outcome of the sectioning process. Appropriate wafering blade speeds are selected based on the hardness, density, and brittleness of materials to ensure that cuts are both efficient and precise.
RPM Selection for Different Material Types
Cutting/Sectioning Hard and Dense Materials:
-
Elevated Speeds for Hard Materials: For hard and dense materials like Silicon Carbide, high RPMs are necessary. The increased speed allows the wafering blade to maintain a consistent cutting action, helping to overcome the material's resistance.
-
Optimized Blade Performance: At higher RPMs, the centrifugal force helps to stabilize the blade, reducing vibrations and enabling a smoother cut. The enhanced momentum at these speeds also assists in clearing the cut material (swarf) from the cutting path, preventing clogging and maintaining a consistent cutting rate.
Handling Brittle Materials:
-
Reduced Speeds for Brittleness: Brittle materials such as silicon wafers and gallium arsenide require a more delicate approach, achieved by operating at lower RPMs. This approach minimizes the risk of inducing microcracks or causing fractures that could compromise the structural integrity of the material.
-
Controlled Cutting Process: Low RPMs allow for greater control and precision, ensuring that the inherent brittleness of the material is accommodated, and damage during the sectioning process is minimized.
Equipment Capabilities and RPM Ranges
Low-Speed Saws:
-
RPM Range and Applications: Low-speed saws, with RPM capabilities ranging from 0 to 600, are ideal for materials that are susceptible to damage from high-speed operations. They allow for fine control of the cutting process and are particularly useful for precision applications in research and development settings where sample integrity is crucial.
-
Customized Cutting Conditions: By limiting the speed, low-speed saws can offer more controlled cutting conditions, which can be essential when dealing with layered materials or composites that might react differently at various depths of the cut.
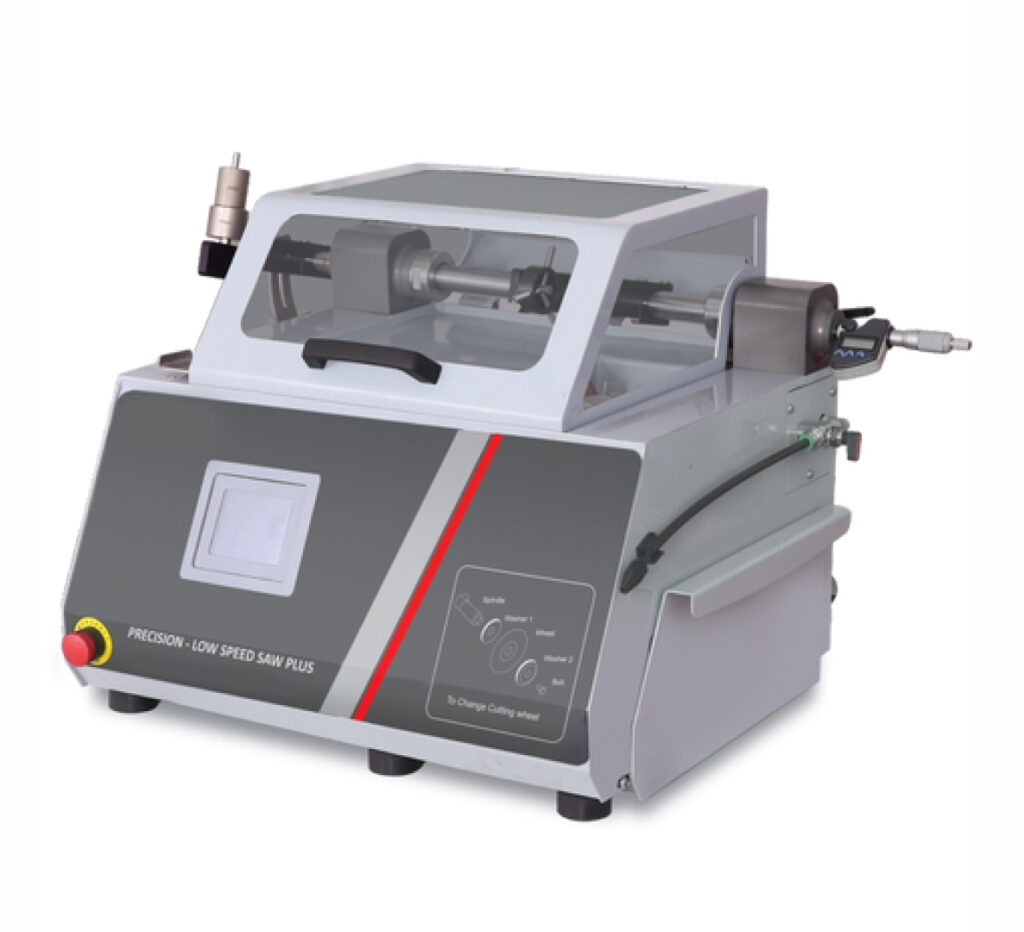
High-Speed Saws:
-
Extended RPM Spectrum: High-speed saws expand the RPM range up to 6,000, providing a broad spectrum of cutting conditions to match a wider variety of materials. This flexibility is key in industrial applications where different materials require rapid throughput without sacrificing cut quality.
-
Versatility and Efficiency: The ability to operate at high speeds offers efficiency and versatility, making high-speed saws suitable for bulk material processing, quick sectioning for quality control, or when preparing samples for further analysis.
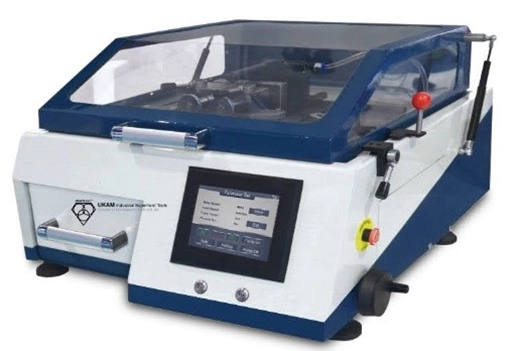
Balancing RPM with Cutting Requirements
-
Material Assessment: A thorough assessment of the material’s properties is necessary to determine the appropriate blade speed. Factors such as material hardness, thermal sensitivity, and expected finish must all be considered.
-
Wafering Blade Specification Alignment: Blade specifications, including diamond grit size and bond hardness, should be aligned with the chosen RPM range to maximize cutting performance and blade life.
-
Safety Considerations: When operating at higher speeds, safety becomes a paramount concern. Proper safety mechanisms and operator training must be in place to handle the increased risk associated with high-speed cutting.
Wafering Blade Relief
Wafering blade relief, along with the distance between the steel core and the diamond section, plays a significant role in the overall effectiveness of these precision blades. Relief in a wafering blade creates a distinct separation between the cutting edge and the steel core, which contributes to improved performance in several ways. This relief design reduces friction, allowing the blade to cut through materials more efficiently while generating less heat. This reduction in heat is crucial, as it minimizes the risk of blade warping or material damage during high-speed cutting.
Moreover, the relief helps in clearing debris that is generated during the cutting process. With less surface contact between the blade's steel core and the material, debris can be more easily cleared away, preventing clogging and ensuring smoother, uninterrupted cuts. The design also contributes to improved cutting speed, making these blades highly effective for industrial applications where precision and efficiency are paramount.
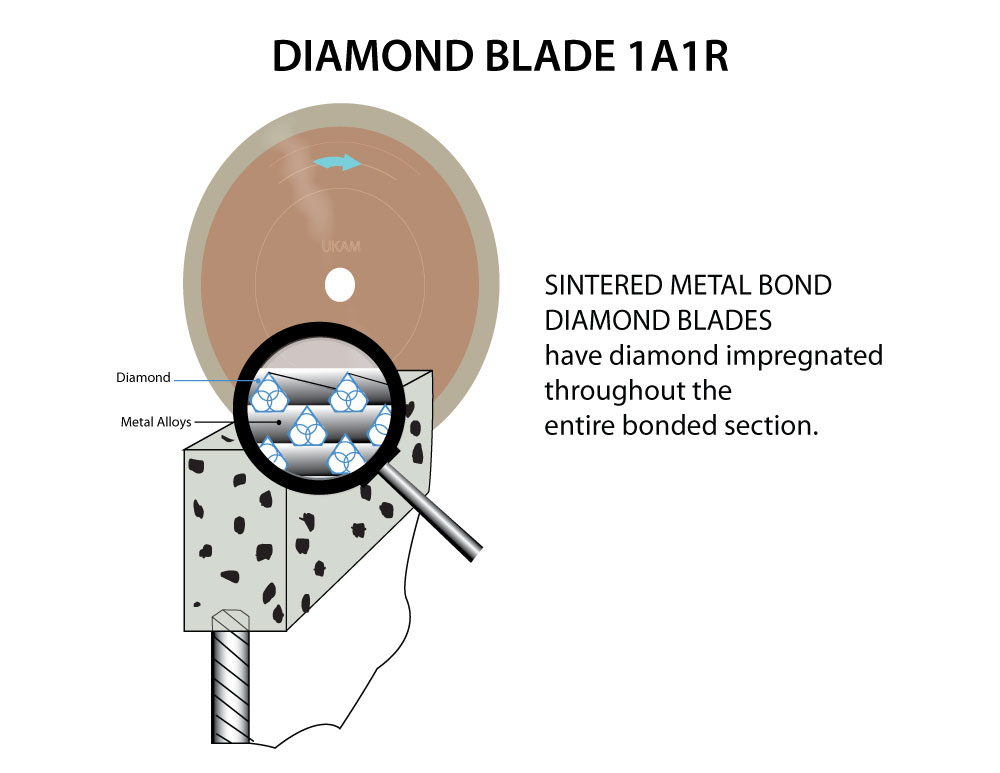
The distance between the blade's steel core and its diamond section is another key aspect of blade design. A thicker core provides greater rigidity, reducing the likelihood of blade wobbling or deflection during use. This stability is critical when precision is essential. However, a thicker core can also lead to increased friction, impacting the blade's performance by generating more heat. The diamond section's width plays a complementary role; a wider diamond section often provides greater stability and cutting accuracy, while a narrower section can be beneficial for delicate or intricate cuts.
Finding the right balance between the thickness of the steel core and the width of the diamond section is key. A smooth transition between these two components ensures even stress distribution, which reduces the risk of blade fracture and contributes to longer blade life. This balance also affects cutting precision. A well-designed core-to-diamond section transition allows for precise, clean cuts with minimal material loss, which is crucial in applications where accuracy and efficiency are critical.
Wafering Blade Inside Diameter / Arbor Size
The inside diameter of a diamond wafering blade is a critical aspect of its design, impacting the wafering blades compatibility with cutting equipment, stability during operation, and overall performance. This component refers to the central hole or arbor where the blade is mounted onto the cutting machine's spindle or shaft. Understanding the significance of the inside diameter helps ensure that the wafering blade is used effectively and safely. Here's a detailed exploration of the inside diameter of diamond wafering blades.
Purpose of the Inside Diameter
The inside diameter serves several essential purposes:
-
Mounting and Alignment: The inside diameter allows the wafering blade to be securely mounted onto the cutting machine's spindle or shaft. This ensures proper alignment and stability during operation, reducing vibration and potential blade wobble.
-
Blade Compatibility: The size of the inside diameter determines which cutting machines the wafering blade can be used with. It must match the spindle size of the machine to ensure a secure fit and reliable performance.
-
Rotation and Cutting Speed: A proper fit between the blades inside diameter and the cutting machine's spindle is crucial for maintaining accurate rotation and cutting speed. An ill-fitting wafering blade can lead to slippage, uneven cuts, or safety hazards.
Standard Sizes for Inside Diameter
Diamond & cbn wafering blades come in various standard inside diameters to accommodate different cutting machines and applications. Common sizes include:
-
1/2 inch (12.7 mm): A common size for smaller cutting machines used in laboratories or metallographic applications.
-
1 inch (25.4 mm): Often used in larger industrial cutting machines.
-
1.25” (31.75mm) – used for high speed and larger sectioning/laboratory & industrial saws
-
32mm – used for high speed and larger sectioning/laboratory & industrial saws
-
Other Sizes: Depending on the manufacturer and specific cutting machine requirements, other inside diameters may be available, such as 5/8 inch (15.875 mm) or 3/4 inch (19.05 mm).
Factors Influencing Inside Diameter Selection
When selecting a diamond or cbn wafering blade, the inside diameter should be chosen based on several factors:
-
Cutting Machine Compatibility: The inside diameter must match the cutting machine's spindle size for a secure and stable fit. Using the wrong size can lead to improper mounting and potential safety risks.
-
Blade Stability and Performance: A proper fit ensures blade stability, reducing vibration and ensuring consistent cutting performance. An incorrect inside diameter can cause wobbling, leading to uneven cuts or blade damage.
-
Application Requirements: The inside diameter size should also align with the specific requirements of the cutting application. For example, smaller inside diameters are typically used in precision applications, while larger diameters may be required for industrial tasks.
Considerations for Inside Diameter
To ensure the correct inside diameter for a diamond wafering blade, consider the following:
-
Check Machine Specifications: Refer to the cutting machine's specifications to determine the appropriate inside diameter size.
-
Use Appropriate Adapters: If necessary, use adapters to fit the blade's inside diameter to the machine's spindle. However, ensure that these adapters are designed for the specific application to maintain stability.
-
Secure Mounting: Properly mount the blade onto the cutting machine's spindle, ensuring a tight and secure fit. Double-check for any wobbling or instability before operation.
Wafering Blade Coatings & Physical Appearance
The surface treatment of the steel core in diamond wafering blades is an essential factor that contributes to the blade's performance, durability, corrosion resistance, and physical appearance. Various surface treatments are used to protect the core from environmental factors, improve its wear resistance, and enhance the bonding between the core and the diamond section. This leads to a longer-lasting wafering blade and more consistent cutting performance. Here's a detailed exploration of core surface treatment methods and their impact on diamond wafering blades.
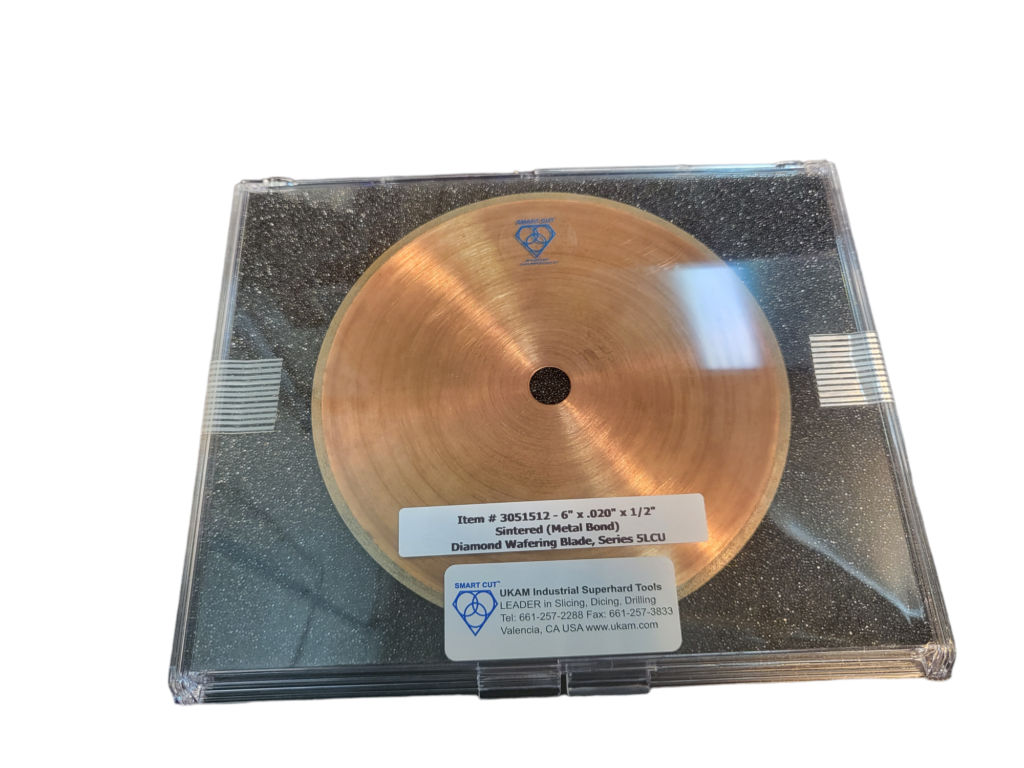
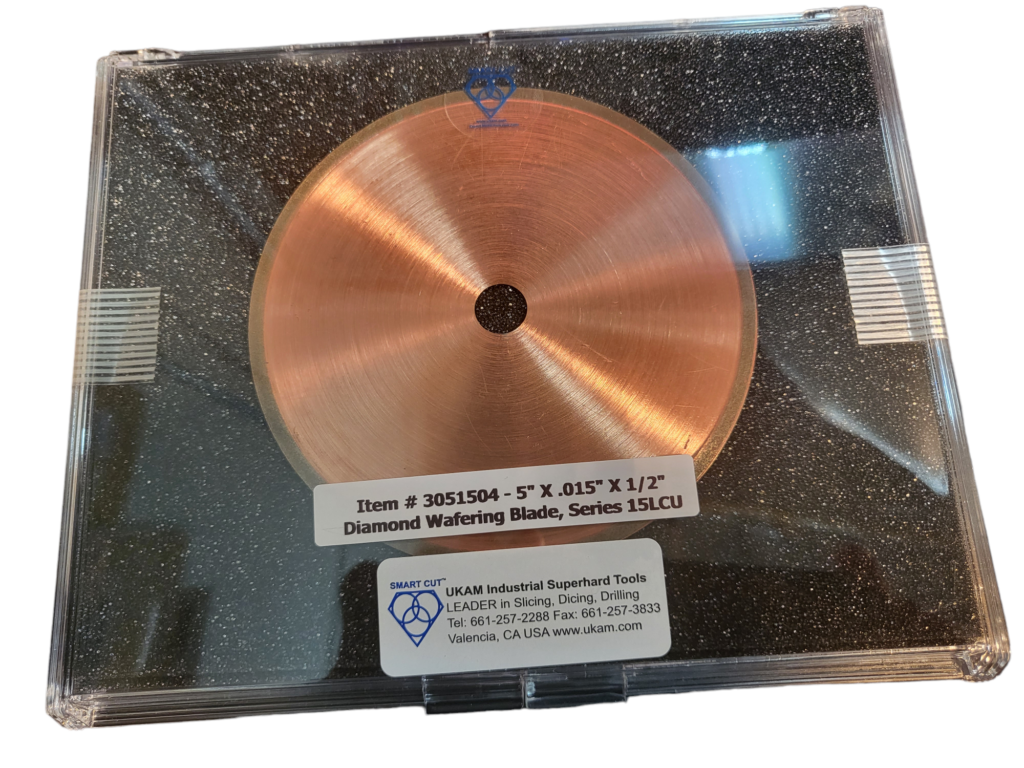
Anti-Corrosion Coatings
Anti-corrosion coatings are applied to steel cores to protect them from rust and oxidation. These coatings are especially important in environments with high humidity or exposure to corrosive substances. Common anti-corrosion coatings include:
-
Nickel Coating: Nickel provides a hard, corrosion-resistant surface. It is often applied to steel cores to enhance durability and protect against rust, particularly in industrial settings where the blade may encounter corrosive chemicals or moisture.
-
Bronze/Copper Coating – there are multiple advantages of using this type of coating bronze/Copper coatings on the core of diamond wafering blades can improve the bonding of diamond particles. This enhanced bonding ensures a stable structure, reducing the risk of the abrasive detaching from the core during cutting operations. The anti-corrosion property of copper-based coatings minimizes rust and oxidation, ensuring consistent performance over time. Copper is an excellent conductor of heat, allowing blades with copper coatings to dissipate heat more effectively during cutting. This is beneficial in sample preparation, where excessive heat can damage the material being cut. Copper-based coatings can also contribute to the wear resistance of the blade. While the primary wear-resistant component is the diamond abrasive, copper coatings provide additional resilience against abrasive environments.
Benefits of Core Surface Treatment
The various core surface treatments offer several benefits:
-
Increased Durability: Anti-corrosion and wear-resistant coatings protect the steel core from environmental factors, extending the blade's lifespan.
-
Enhanced Performance: Surface treatments that improve bonding and reduce friction contribute to more consistent cutting performance and smoother cuts.
-
Reduced Maintenance: A steel core with effective surface treatment requires less maintenance, as it is less prone to rust and wear.
-
Improved Safety: By preventing delamination and ensuring a strong bond between the core and the diamond section, these treatments enhance the blade's safety during operation.
Wafering Blade Core Steel
The steel core in a diamond wafering blade plays a central role in defining the blade's performance, durability, and safety. This backbone component is responsible for providing the necessary structure to support the diamond section and ensure stability during cutting. The specific properties of the steel core, such as hardness, flexibility, and heat resistance, are crucial in determining how the blade behaves under various conditions.
A steel core's hardness is critical for maintaining the blade's structural integrity. A hard core resists deformation and warping, allowing the blade to stay true to its form during cutting. This characteristic is essential for achieving precise cuts, as any deviation in the blade's shape can lead to uneven results and increased wear.
Flexibility in the steel core complements its hardness. While a rigid core is necessary for stability, some degree of flexibility helps absorb shocks and vibrations that occur during cutting. This flexibility reduces the risk of the blade cracking or breaking, contributing to smoother operation and a longer lifespan. The ability to absorb vibrations also enhances the cutting experience by reducing noise and ensuring a smoother cut through the material.
Heat resistance is another key property of the steel core. The cutting process generates significant heat due to friction, and a core that can withstand high temperatures prevents warping or structural damage. A heat-resistant core ensures the blade's durability and maintains cutting precision over time, even during extended periods of use.
Wear resistance in the steel core is equally important. A core with high resistance to wear ensures the blade's longevity, allowing it to maintain its performance over a prolonged period. This wear resistance reduces the need for frequent blade replacements, making the blade more cost-effective in the long run.
The manufacturing process and composition of the steel core are essential factors in achieving the desired properties. The steel alloys used for the core, such as high-carbon steel or stainless steel, are chosen for their strength, flexibility, and heat resistance. The manufacturing process, including techniques like tempering, annealing, and heat treatment, enhances these properties, resulting in a core that meets the demands of various cutting applications.
Precision engineering in the manufacturing process ensures that the steel core is uniform in thickness and free from defects. This precision is critical for maintaining balance and stability during cutting. A uniform core helps prevent vibration and wobbling, which could otherwise lead to uneven cuts or blade damage.
The quality of the steel core directly influences the blade's performance and safety. A stable core ensures consistent cuts and reduces the risk of blade failure during operation. A high-quality core also contributes to operator safety by minimizing the chance of blade breakage. The balance of hardness, flexibility, heat resistance, and wear resistance in the steel core is what makes diamond wafering blades reliable and durable for a wide range of cutting applications.
Core Hardness and Strength
The hardness and strength of the steel core are fundamental to the performance and durability of diamond wafering blades. These characteristics determine the core's ability to withstand the stresses of cutting, its resistance to deformation, and its ability to support the diamond section without flexing or warping. A high-quality steel core with appropriate hardness and strength is essential for achieving consistent and reliable cutting performance. Here's a comprehensive look at core hardness and strength and their importance in diamond wafering blades.
Core Hardness
Hardness in the context of a steel core refers to its resistance to deformation, scratching, or indentation. In diamond wafering blades, a hard steel core provides several key advantages:
-
Structural Integrity: A hard core maintains its shape during cutting, preventing warping or bending under pressure. This integrity is crucial for achieving precise cuts and reducing blade wobble.
-
Resistance to Wear: Harder steel cores are more resistant to wear and abrasion, which contributes to the blade's longevity. This resistance is particularly important in high-stress cutting applications, where the core is subjected to significant friction.
-
Stable Diamond Bonding: A hard core provides a stable surface for bonding with the diamond section. This stability reduces the risk of delamination or diamond particle loss, ensuring consistent cutting performance.
-
Core Thickness and Design: Thicker cores generally require more hardness to maintain structural integrity. Design elements such as reinforcement points or patterns can also impact hardness requirements.
Core Strength
Strength refers to the steel core's ability to withstand applied forces without breaking or deforming. A strong core is vital for ensuring the blade's safety and reliability during cutting. Core strength contributes to several aspects of blade performance:
-
Resistance to Breakage: A strong core can handle the forces generated during cutting without fracturing or breaking. This resistance is essential for operator safety and blade reliability.
-
Flexibility and Toughness: While strength is critical, some degree of flexibility in the core allows it to absorb shocks and vibrations during cutting. This balance between strength and flexibility reduces the risk of blade breakage and contributes to smoother cutting action.
-
Support for Diamond Section: A strong core provides the necessary support for the diamond section, ensuring that it remains securely attached during cutting. This support helps maintain a consistent cutting edge and reduces the risk of blade failure.
Factors Influencing Core Hardness and Strength
Several factors influence the hardness and strength of a steel core:
-
Material Composition: The choice of steel alloy plays a significant role in determining hardness and strength. High-carbon steel and stainless steel are commonly used for their durability and resistance to wear.
-
Heat Treatment: Heat treatment processes, such as tempering and annealing, can enhance the core's hardness and strength. These processes involve controlled heating and cooling to achieve the desired properties.
-
Core Design and Manufacturing: The design of the steel core, including its thickness and reinforcement points, can impact its strength. A well-engineered core with uniform thickness provides greater stability and resistance to deformation.
Importance of Hardness and Strength in Wafering Blades
The hardness and strength of the steel core are critical for achieving consistent and reliable cutting performance. A hard and strong core provides the following benefits:
The hardness range of the steel core in diamond wafering blades is a crucial parameter, affecting the blade's performance, durability, and resistance to deformation. Hardness indicates the steel's resistance to indentation, scratching, or deformation and is typically measured using the Rockwell or Vickers hardness scales. The exact hardness range for the steel core in diamond wafering blades can vary depending on the blade's intended use, the type of steel alloy, and the manufacturing process. However, there are general trends in the typical hardness range for these applications.
Rockwell Hardness Scale
The Rockwell hardness scale is commonly used to measure the hardness of steel cores. It uses a diamond cone or a steel ball to indent the material, with the hardness rating based on the depth of the indentation. The scale has different scales for different materials, with "HRC" being one of the most common scales used for measuring steel hardness.
For diamond wafering blades, the typical Rockwell hardness range for the steel core is:
-
Between 40 HRC and 60 HRC: This range provides a balance between hardness and flexibility. A core within this range is hard enough to resist wear and deformation, yet flexible enough to absorb shocks and vibrations during cutting.
A core with a hardness below this range might lack the required resistance to wear, leading to faster degradation. Conversely, a core with hardness above this range might be too brittle, increasing the risk of breakage or cracking under stress.
Wafering Blade Possible Core Types:
Steel
Steel cores are commonly used in wafering blades for general-purpose cutting in metallography. They offer a good balance of hardness, strength, and flexibility, suitable for a wide range of materials. Steel-core blades are often used for sectioning metals and other relatively easy-to-cut materials, making them a cost-effective choice for routine metallographic work. These blades are typically used in laboratories with controlled environments, where corrosion resistance is not a significant concern.
Stainless Steel
Stainless steel cores are ideal for metallographic applications where corrosion resistance is essential. These cores are resistant to rust and oxidation, making them suitable for environments with high humidity or exposure to corrosive substances. Stainless steel-core blades are often used in metallography labs where samples may come into contact with chemicals or moisture during preparation. They are also preferred for sectioning samples that require a higher level of cleanliness to avoid contamination.
Bronze
Bronze cores in diamond wafering blades offer excellent flexibility and thermal conductivity. While they are softer than steel, their flexibility allows for smoother cuts, reducing the risk of material deformation. Bronze-core blades are often used in metallographic applications where precise, delicate cuts are required, such as preparing thin sections of soft metals or other easily deformable materials. The high thermal conductivity of bronze helps manage heat during cutting, reducing the risk of sample damage.
Non-Magnetic Properties
Bronze, an alloy primarily composed of copper with smaller amounts of other elements like tin, is inherently non-magnetic. This property is particularly valuable in metallography and geological sample preparation when dealing with materials that are sensitive to magnetic fields.
Advantages for Metallography
In metallography, non-magnetic bronze-core blades are advantageous for the following reasons:
-
Preventing Magnetic Interference: In certain metallographic analyses, such as electron microscopy or other techniques involving magnetic fields, a non-magnetic blade is essential to avoid interference. Magnetic materials can distort the results, leading to inaccurate readings.
-
Protecting Sensitive Instruments: When preparing samples for analysis using instruments sensitive to magnetic fields, such as scanning electron microscopes (SEM) or magnetic resonance imaging (MRI), a non-magnetic blade helps prevent damage to the equipment. This protection ensures that the samples can be analyzed without compromising the instruments' functionality.
-
Maintaining Sample Integrity: Some geological and metallographic samples contain minerals or metals with magnetic properties. Using a non-magnetic bronze-core blade ensures that these properties remain unaltered during cutting, preserving the sample's natural characteristics for accurate analysis.
Applications in Geological Sample Preparation
In geological sample preparation, non-magnetic bronze-core blades are particularly useful when dealing with rock and mineral samples containing magnetic materials or requiring analysis that might be disrupted by magnetic interference. These blades allow for clean, precise cuts without affecting the sample's magnetic properties.
Steel with Memory
Steel with memory, or shape-memory steel, is valuable in metallographic applications that require a combination of flexibility and stability. These cores can return to their original shape after deformation, allowing them to absorb shocks and vibrations during cutting. This property is useful in metallography for preparing samples with complex shapes or when cutting under variable conditions. Steel with memory-core blades are ideal for applications where the blade must withstand high stress without permanent deformation. These are typically used for nickel bond wafering blades
High-Carbon Steel
High-carbon steel cores are preferred for metallographic applications that require high hardness and strength. These cores are highly durable and resistant to wear, making them suitable for cutting hard metals and materials that demand consistent performance over time. High-carbon steel-core blades are often used for sectioning samples in industrial metallography, where the blades must endure high stress and frequent use. They are also ideal for preparing samples for hardness and tensile testing, where precision and durability are crucial.
Tungsten Carbide
Tungsten carbide cores are known for their exceptional hardness and wear resistance, making them ideal for metallographic applications that involve cutting hard and abrasive materials. Their durability and high precision make them suitable for applications requiring intricate cuts and minimal material deformation. Although very costly, tungsten carbide-core blades are valued for their longevity and reliability in ultra demanding metallographic environments.
Fully Sintered (Metal Bond) Wafering Blades
Fully Sintered (Metal Bond) diamond wafering blades are fully sintered from OD to ID of the blade. Meaning they have diamonds completely impregnated through the blade. Unlike standard diamond & cbn wafering blades with steel core and diamond section. Instead of having a steel core and small diamond bond edge (usually 1/8″/3.2mm). The diamond edge is all the way through the blade, from its Outside Diameter to Inside Diameter of the blade. You can use until the entire Outside Diameter of the blade is consumed.
These types of blades may last as much as 3 to 4 standard blades with steel core put together. Since the entire blade is diamond and same thickness from OD to ID (with no thinner steel core to flex, warp, or loose its flatness). The fully sintered (metal bond) blades is more rigid. Providing minimum heat generation and stress to material being cut. This allows for thinner kerf thicknesses that would not be normally possible with wafering blades made with steel core, longer blade life, better cut quality and more rigidity. These are an excellent choice for sectioning large variety of ultra-hard and brittle materials, delicate samples, crystals, teeth, bones, composites, metals, and much more.
-
Consistent Cutting Performance: A stable and strong core ensures that the blade remains rigid during cutting, leading to precise and consistent cuts.
-
Extended Blade Lifespan: Resistance to wear and breakage contributes to the blade's longevity, reducing the need for frequent replacements.
-
Enhanced Safety: A strong core reduces the risk of blade breakage, ensuring a safer cutting experience for the operator and the surrounding environment.
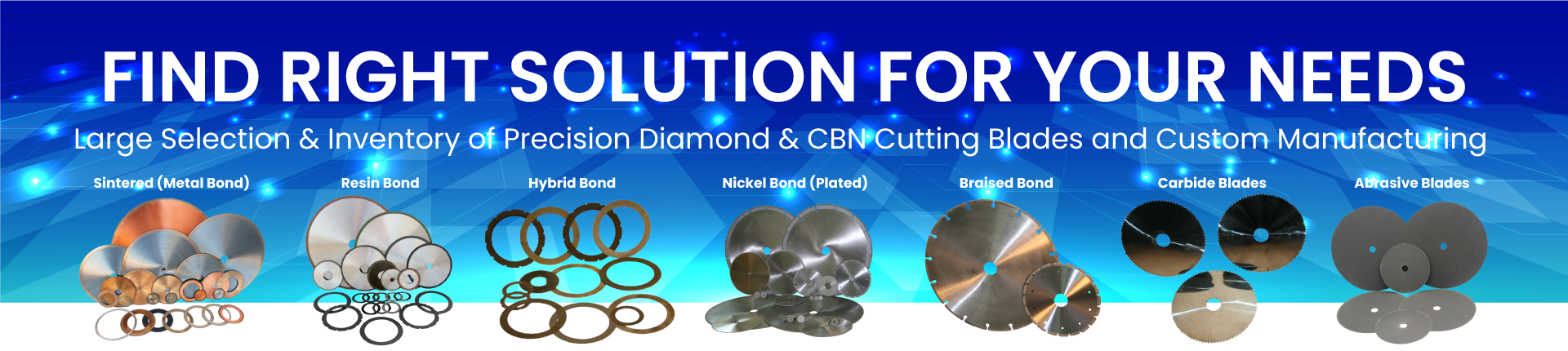